Acids
acid base and salt: As we delve into the world of chemistry, one of the most fundamental concepts we encounter is that of acids. These substances play a crucial role in various chemical reactions and are ubiquitous in our daily lives. In this section, we’ll explore the nature of acids, their properties, and their significance in both scientific and practical contexts.
Definition and Characteristics of Acids
Acids are chemical compounds that exhibit specific properties when dissolved in water or other solvents. The key characteristics that define acids include:
- Sour taste
- Ability to react with metals to produce hydrogen gas
- Capacity to turn blue litmus paper red
- Tendency to donate protons (H+ ions) in chemical reactions
These properties form the basis of our understanding of acids and their behavior in various chemical processes.
Theories of Acids
Over time, scientists have developed several theories to explain the behavior of acids. Let’s examine the three most prominent theories:
Arrhenius Theory
Proposed by Svante Arrhenius in 1884, this theory defines acids as substances that increase the concentration of hydrogen ions (H+) when dissolved in water. According to this theory, an acid dissociates in water to produce H+ ions.
Example: HCl → H+ + Cl-
Brønsted-Lowry Theory
Developed independently by Johannes Brønsted and Thomas Lowry in 1923, this theory expanded on the Arrhenius concept. It defines acids as proton donors in chemical reactions. This theory allows for a broader understanding of acid-base reactions, including those that occur in non-aqueous solutions.
Example: HCl + H2O → H3O+ + Cl-
Lewis Theory
Proposed by Gilbert Lewis in 1923, this theory further broadened the concept of acids. According to Lewis, an acid is an electron pair acceptor. This definition encompasses reactions that don’t involve proton transfer and applies to a wider range of chemical reactions.
Example: BF3 + NH3 → F3B-NH3
Here’s a comparison of these theories in a Markdown table:
Theory | Definition of Acid | Scope |
---|---|---|
Arrhenius | H+ donor in aqueous solutions | Limited to water-based reactions |
Brønsted-Lowry | Proton donor | Includes non-aqueous solutions |
Lewis | Electron pair acceptor | Broadest definition, includes non-proton transfer reactions |
Types of Acids
Acids can be classified in various ways based on their strength, composition, or other properties. Here are some common classifications:
Strong vs. Weak Acids
- Strong acids: Completely dissociate in water (e.g., HCl, H2SO4, HNO3)
- Weak acids: Partially dissociate in water (e.g., CH3COOH, H2CO3, H3PO4)
Monoprotic vs. Polyprotic Acids
- Monoprotic: Can donate one proton per molecule (e.g., HCl, HNO3)
- Diprotic: Can donate two protons per molecule (e.g., H2SO4, H2CO3)
- Triprotic: Can donate three protons per molecule (e.g., H3PO4)
Organic vs. Inorganic Acids
- Organic acids: Contain carbon (e.g., CH3COOH, HCOOH)
- Inorganic acids: Do not contain carbon (e.g., HCl, H2SO4, HNO3)
Oxyacids vs. Hydracids
- Oxyacids: Contain oxygen (e.g., H2SO4, HNO3)
- Hydracids: Do not contain oxygen (e.g., HCl, HF)
The pH Scale and Acid Strength
The pH scale is a crucial tool for measuring the acidity or basicity of a solution. It ranges from 0 to 14, with 7 being neutral. Values below 7 indicate acidity, while values above 7 indicate basicity.
The pH scale is logarithmic, meaning each unit change represents a tenfold difference in H+ ion concentration. For instance, a solution with a pH of 4 is ten times more acidic than a solution with a pH of 5.
Acid strength is directly related to the ability of an acid to dissociate and produce H+ ions in solution. The stronger the acid, the lower its pH value. Here’s a brief overview:
- Strong acids: pH 0-3 (e.g., HCl, H2SO4)
- Weak acids: pH 3-7 (e.g., CH3COOH, H2CO3)
- Neutral: pH 7 (e.g., pure water)
- Weak bases: pH 7-11
- Strong bases: pH 11-14
Common Household Acids
Acids are not just confined to chemistry laboratories; they are present in many household items and foods. Here are some common household acids:
- Citric Acid (C6H8O7): Found in citrus fruits like lemons and oranges
- Acetic Acid (CH3COOH): The main component of vinegar
- Tartaric Acid (C4H6O6): Present in grapes and used in baking powder
- Ascorbic Acid (C6H8O6): Also known as Vitamin C, found in many fruits and vegetables
- Carbonic Acid (H2CO3): Found in carbonated beverages
Chemical Reactions Involving Acids
Acids participate in various chemical reactions that are fundamental to many processes in chemistry and biology. Some key reactions include:
Acid-Base Reactions (Neutralization)
These reactions occur between acids and bases, resulting in the formation of water and a salt.
Example: HCl + NaOH → NaCl + H2OAcid-Metal Reactions
Many metals react with acids to produce hydrogen gas and a metal salt.
Example: 2HCl + Zn → ZnCl2 + H2Acid-Carbonate Reactions
Acids react with carbonates to produce carbon dioxide, water, and a salt.
Example: 2HCl + CaCO3 → CaCl2 + H2O + CO2Acid-Oxide Reactions
Acids can react with metal oxides to form water and a salt.
Example: 2HCl + CuO → CuCl2 + H2O
Industrial and Biological Applications of Acids
Acids play crucial roles in various industrial processes and biological systems:
Industrial Applications:
- Production of fertilizers (e.g., sulfuric acid in the production of phosphate fertilizers)
- Manufacturing of plastics and synthetic fibers
- Metal processing and cleaning
- Food preservation and pH adjustment in food products
- Production of pharmaceuticals
Biological Applications:
- Hydrochloric acid in stomach aids digestion
- Amino acids are the building blocks of proteins
- Nucleic acids (DNA and RNA) carry genetic information
- Fatty acids are important components of cell membranes
Environmental Impact of Acids
While acids are essential for many processes, they can also have significant environmental impacts:
Acid Rain:
Formed when sulfur dioxide and nitrogen oxides react with water in the atmosphere, acid rain can harm ecosystems, damage buildings, and affect human health.Ocean Acidification:
Increased CO2 levels in the atmosphere lead to higher absorption by oceans, forming carbonic acid and lowering the pH of seawater. This affects marine ecosystems, particularly organisms with calcium carbonate shells or skeletons.Soil Acidification:
Overuse of nitrogen-based fertilizers and acid rain can increase soil acidity, affecting plant growth and soil ecosystem health.
Safety Considerations When Handling Acids
Given their reactive nature, proper handling of acids is crucial for safety:
Personal Protective Equipment (PPE):
- Always wear safety goggles, gloves, and appropriate protective clothing
- Use a fume hood when working with volatile acids
Dilution:
- Always add acid to water, never water to acid, to prevent dangerous splashing
- Dilute concentrated acids before use
Storage:
- Store acids in proper containers away from bases and reactive metals
- Ensure proper labeling and segregation of different types of acids
Spill Management:
- Have neutralizing agents (like sodium bicarbonate) readily available
- Follow proper spill cleanup procedures
First Aid:
- Know the location of safety showers and eyewash stations
- Immediately flush affected areas with water in case of acid contact
Future Directions in Acid Research and Applications
As our understanding of acids continues to evolve, several exciting areas of research and application are emerging:
Green Chemistry:
Developing eco-friendly acids and acid-based processes to reduce environmental impactAdvanced Materials:
Using acids in the synthesis of novel materials like graphene and nanotubesEnergy Storage:
Exploring the role of acids in advanced battery technologiesBiomedical Applications:
Investigating the use of acids in drug delivery systems and tissue engineeringSustainable Agriculture:
Developing acid-based fertilizers and soil treatments for improved crop yield and reduced environmental impact
As we conclude our exploration of acids, it’s clear that these substances are fundamental to countless chemical processes and applications. From the food we eat to the materials we use, acids play a vital role in shaping our world. Their properties, reactions, and impacts continue to be areas of intense study and innovation in chemistry and related fields.
Now that we have covered acids in detail, let’s move on to explore their chemical counterparts: bases. Understanding the relationship between acids and bases is crucial for a comprehensive grasp of chemical reactions and their applications in various fields.
Bases
Now that we’ve explored acids, let’s delve into the world of bases. Bases are equally important in chemistry and play a crucial role in many chemical reactions and everyday applications.
Definition and Characteristics of Bases
Bases are substances that can accept protons (H+ ions) or donate electron pairs in chemical reactions. They are the counterparts to acids and exhibit several distinctive properties:
- Bitter taste
- Slippery or soapy feel
- Ability to turn red litmus paper blue
- pH values greater than 7 on the pH scale
Theories of Bases
To fully understand bases, it’s essential to examine the three main theories that define them:
Arrhenius Theory
The Arrhenius theory, proposed by Svante Arrhenius in 1884, defines a base as a substance that dissociates in water to produce hydroxide ions (OH-). This theory is limited to aqueous solutions and cannot explain the basic behavior of substances that don’t contain hydroxide ions.
Examples of Arrhenius bases:
- Sodium hydroxide (NaOH)
- Potassium hydroxide (KOH)
- Calcium hydroxide (Ca(OH)2)
Brønsted-Lowry Theory
The Brønsted-Lowry theory, developed independently by Johannes Brønsted and Thomas Lowry in 1923, expands on the Arrhenius theory. It defines a base as a proton (H+) acceptor. This theory can explain the basic behavior of substances that don’t contain hydroxide ions and is applicable to non-aqueous solutions.
Examples of Brønsted-Lowry bases:
- Ammonia (NH3)
- Carbonate ion (CO3^2-)
- Bicarbonate ion (HCO3^-)
Lewis Theory
The Lewis theory, proposed by Gilbert N. Lewis in 1923, provides the most comprehensive definition of bases. According to this theory, a base is an electron pair donor. This definition encompasses both the Arrhenius and Brønsted-Lowry theories and can explain the basic behavior of substances in reactions that don’t involve proton transfer.
Examples of Lewis bases:
- Ammonia (NH3)
- Ethers (R-O-R)
- Amines (R-NH2)
Types of Bases
Bases can be classified into several categories based on their composition and properties:
- Strong bases
- Weak bases
- Alkali metal hydroxides
- Alkaline earth metal hydroxides
- Organic bases
Let’s explore each of these types in detail:
Strong Bases
Strong bases are those that completely dissociate in aqueous solutions, releasing all of their hydroxide ions. They have a high pH (usually 13-14) and are highly corrosive.
Examples of strong bases:
- Sodium hydroxide (NaOH)
- Potassium hydroxide (KOH)
- Lithium hydroxide (LiOH)
Weak Bases
Weak bases only partially dissociate in aqueous solutions, releasing a limited number of hydroxide ions. They have a lower pH compared to strong bases (usually 8-11) and are less corrosive.
Examples of weak bases:
- Ammonia (NH3)
- Methylamine (CH3NH2)
- Pyridine (C5H5N)
Alkali Metal Hydroxides
These are bases formed by combining alkali metals (Group 1 elements) with hydroxide ions. They are all strong bases and highly soluble in water.
Examples of alkali metal hydroxides:
- Sodium hydroxide (NaOH)
- Potassium hydroxide (KOH)
- Rubidium hydroxide (RbOH)
Alkaline Earth Metal Hydroxides
These bases are formed by combining alkaline earth metals (Group 2 elements) with hydroxide ions. They are generally less soluble in water compared to alkali metal hydroxides.
Examples of alkaline earth metal hydroxides:
- Calcium hydroxide (Ca(OH)2)
- Magnesium hydroxide (Mg(OH)2)
- Barium hydroxide (Ba(OH)2)
Organic Bases
Organic bases are carbon-containing compounds that can accept protons or donate electron pairs. They are often weak bases and play important roles in biochemistry and organic synthesis.
Examples of organic bases:
- Ethylamine (C2H5NH2)
- Aniline (C6H5NH2)
- Pyridine (C5H5N)
Properties of Bases
Bases exhibit several characteristic properties that distinguish them from other substances:
- pH value: Bases have a pH greater than 7, with strong bases having pH values closer to 14.
- Electrical conductivity: Aqueous solutions of bases conduct electricity due to the presence of ions.
- Reaction with acids: Bases neutralize acids to form salts and water.
- Reaction with metals: Some bases can react with certain metals to form salts and hydrogen gas.
- Reaction with organic compounds: Bases can catalyze various organic reactions, such as hydrolysis and saponification.
Let’s explore some of these properties in more detail:
pH Scale and Basicity
The pH scale is a logarithmic measure of the hydrogen ion concentration in a solution. For bases, the pH is determined by the hydroxide ion concentration. The relationship between pH and pOH (a measure of hydroxide ion concentration) is:
pH + pOH = 14
As the concentration of hydroxide ions increases, the pH increases, and the solution becomes more basic. Here’s a table showing the relationship between pH, pOH, and the concentrations of H+ and OH- ions:
pH | pOH | [H+] (mol/L) | [OH-] (mol/L) | Nature of solution |
---|---|---|---|---|
7 | 7 | 1 × 10^-7 | 1 × 10^-7 | Neutral |
8 | 6 | 1 × 10^-8 | 1 × 10^-6 | Slightly basic |
10 | 4 | 1 × 10^-10 | 1 × 10^-4 | Basic |
12 | 2 | 1 × 10^-12 | 1 × 10^-2 | Strongly basic |
14 | 0 | 1 × 10^-14 | 1 | Very strongly basic |
Electrical Conductivity
Bases conduct electricity in aqueous solutions due to the presence of mobile ions. When a base dissociates in water, it produces hydroxide ions (OH-) and positively charged cations. These ions can carry an electric current through the solution.
The strength of a base affects its electrical conductivity:
- Strong bases: High electrical conductivity due to complete dissociation
- Weak bases: Lower electrical conductivity due to partial dissociation
Common Household Bases
Many common household products contain bases. Understanding these everyday bases can help us appreciate their importance in our daily lives:
- Baking soda (sodium bicarbonate, NaHCO3): A weak base used in baking and cleaning
- Soap: Contains alkaline compounds that help remove dirt and oils
- Ammonia: A weak base used in household cleaners
- Milk of magnesia (magnesium hydroxide, Mg(OH)2): An antacid used to treat indigestion
- Lye (sodium hydroxide, NaOH): A strong base used in some drain cleaners and soap making
Applications of Bases
Bases have numerous applications in industry, agriculture, and everyday life:
Neutralization of acids: Bases are used to neutralize acids in various processes, such as treating acid spills or adjusting soil pH.
Cleaning agents: Many cleaning products contain bases to help remove grease and grime.
Food industry: Bases are used in food processing for pH adjustment and as leavening agents.
Pharmaceutical industry: Bases are used in the production of various medications and as active ingredients in antacids.
Water treatment: Bases are used to adjust the pH of water in treatment plants and swimming pools.
Textile industry: Bases are used in the production of dyes and in various textile processing steps.
Paper production: Bases are used in the pulping process to break down wood fibers.
Soap and detergent manufacturing: Bases are key ingredients in the saponification process for making soaps.
Safety Considerations When Handling Bases
While bases are essential in many applications, they can be dangerous if not handled properly. Here are some important safety considerations:
Wear protective equipment: Always use gloves, safety goggles, and appropriate clothing when handling bases.
Proper storage: Store bases in clearly labeled, sealed containers away from acids and other reactive substances.
Dilution: When diluting bases, always add the base to water, never water to the base, to avoid splashing and excessive heat generation.
Neutralization: In case of spills or skin contact, neutralize the base with a mild acid solution (e.g., vinegar) before washing with water.
Ventilation: Work in well-ventilated areas to avoid inhaling fumes from volatile bases.
First aid: Know the appropriate first aid procedures for base exposure, including flushing affected areas with water for at least 15 minutes.
Reactions Involving Bases
Bases participate in various chemical reactions, some of which are fundamental to understanding their behavior and applications:
Neutralization reactions: Bases react with acids to form salts and water. This is the basis for many important processes, including the treatment of acid reflux in the human body.
Example: HCl (acid) + NaOH (base) → NaCl (salt) + H2O (water)
Saponification: Bases catalyze the hydrolysis of esters to produce soaps. This reaction is the foundation of soap manufacturing.
Example: CH3(CH2)16COO(CH2)3CH3 + NaOH → CH3(CH2)16COONa + CH3(CH2)3OH
Precipitation reactions: Some bases can react with metal ions to form insoluble hydroxides, which precipitate out of solution.
Example: Cu^2+ + 2NaOH → Cu(OH)2 (precipitate) + 2Na^+
Gas-forming reactions: Certain bases can react with ammonium salts to produce ammonia gas.
Example: NH4Cl + NaOH → NH3 (gas) + H2O + NaCl
Hydrolysis of salts: When salts of weak acids or weak bases dissolve in water, they undergo hydrolysis, which can result in basic solutions.
Example: Na2CO3 + H2O ⇌ NaHCO3 + NaOH
Base Strength and Dissociation Constants
The strength of a base is determined by its ability to accept protons or donate electron pairs. This strength can be quantified using the base dissociation constant (Kb) or the negative logarithm of Kb (pKb).
For a base B reacting with water:
B + H2O ⇌ BH+ + OH-
The base dissociation constant (Kb) is given by:
Kb = [BH+][OH-] / [B]
The larger the Kb value, the stronger the base. Alternatively, we can use pKb:
pKb = -log10(Kb)
In this case, the smaller the pKb value, the stronger the base.
Here’s a table comparing the Kb and pKb values for some common bases:
Base | Kb | pKb |
---|---|---|
Sodium hydroxide | Very large | < 0 |
Ammonia | 1.8 × 10^-5 | 4.75 |
Methylamine | 4.4 × 10^-4 | 3.36 |
Pyridine | 1.7 × 10^-9 | 8.77 |
Water | 1.0 × 10^-14 | 14 |
Buffer Solutions and Bases
Buffer solutions are important in many biological and chemical processes. They resist changes in pH when small amounts of acids or bases are added. Many buffer solutions involve weak bases and their conjugate acids.
A typical base buffer consists of a weak base and its conjugate acid salt. For example, an ammonia-ammonium chloride buffer:
NH3 + H2O ⇌ NH4+ + OH-
The Henderson-Hasselbalch equation can be used to calculate the pH of a buffer solution:
pH = pKa + log([A-] / [HA])
Where [A-] is the concentration of the conjugate base and [HA] is the concentration of the weak acid.
Environmental Impact of Bases
While bases are essential in many applications, their release into the environment can have significant impacts:
Soil alkalinization: Excessive use of bases in agriculture or industrial runoff can increase soil pH, affecting plant growth and soil microorganisms.
Water pollution: Discharge of basic substances into water bodies can alter aquatic ecosystems, harming fish and other organisms.
Air quality: Some volatile bases can contribute to air pollution and the formation of particulate matter.
Corrosion: Strong bases can corrode metals and damage infrastructure if not properly contained.
To mitigate these impacts, proper handling, storage, and disposal of bases are crucial. Many industries employ neutralization techniques and wastewater treatment to minimize the environmental impact of basic substances.
As we transition to the next section on salts, it’s important to remember that bases play a crucial role in salt formation through neutralization reactions with acids. Understanding the properties and behaviors of bases is essential for comprehending the characteristics and applications of the salts they form.
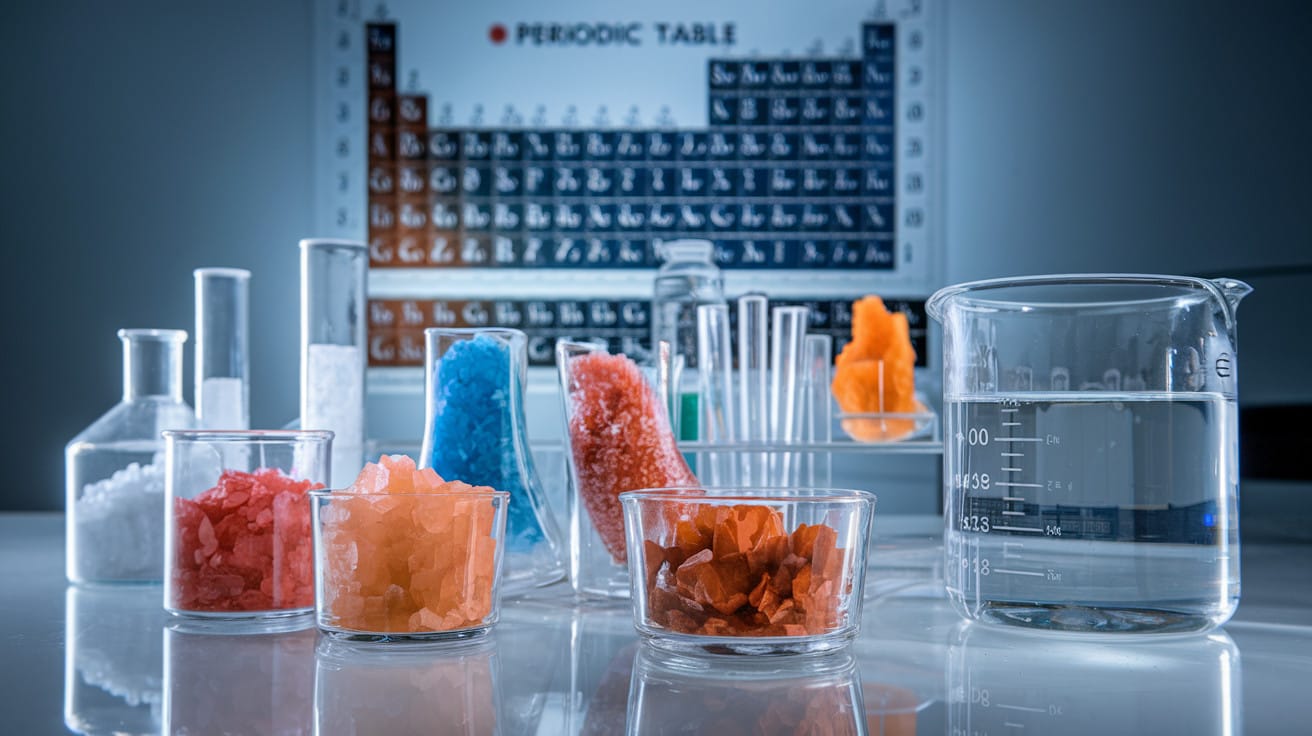
Salts
As we delve into the world of salts, it’s important to understand their fundamental role in chemistry and their interconnection with acids and bases. Salts are ionic compounds that result from the neutralization reaction between an acid and a base. They play a crucial role in various chemical processes and are ubiquitous in our daily lives.
Formation of Salts
Salts are primarily formed through the neutralization reaction between an acid and a base. This process involves the exchange of protons (H+ ions) between the acid and the base, resulting in the formation of water and a salt. The general equation for this reaction can be represented as:
Acid + Base → Salt + Water
For example, when hydrochloric acid (HCl) reacts with sodium hydroxide (NaOH), it forms sodium chloride (NaCl) and water:
HCl + NaOH → NaCl + H2O
This neutralization reaction is a perfect example of salt formation, demonstrating how the hydrogen ion from the acid combines with the hydroxide ion from the base to form water, while the remaining ions combine to form the salt.
Types of Salts
Salts can be classified into various categories based on their composition and properties. Here are some of the main types of salts:
Normal Salts: These are salts formed by the complete neutralization of an acid by a base. Examples include sodium chloride (NaCl) and potassium nitrate (KNO3).
Acid Salts: These salts are formed when an acid is only partially neutralized by a base. They contain replaceable hydrogen atoms. Examples include sodium hydrogen carbonate (NaHCO3) and potassium hydrogen sulfate (KHSO4).
Basic Salts: These are formed when a base is not completely neutralized by an acid. They contain excess hydroxyl ions. Examples include magnesium hydroxide chloride (Mg(OH)Cl) and copper hydroxide sulfate (Cu(OH)2SO4).
Double Salts: These are formed by the combination of two different salts in stoichiometric proportions. Examples include potassium alum (KAl(SO4)2·12H2O) and Mohr’s salt ((NH4)2Fe(SO4)2·6H2O).
Complex Salts: These salts contain complex ions. Examples include potassium ferrocyanide (K4[Fe(CN)6]) and sodium tetraborate (Na2B4O7).
Properties of Salts
Salts exhibit a wide range of properties, which can vary depending on their composition. Here are some general properties of salts:
Physical State: Most salts are solid at room temperature, with crystalline structures.
Solubility: Many salts are soluble in water, but their solubility can vary greatly. Some salts, like silver chloride (AgCl), are practically insoluble in water.
Electrical Conductivity: When dissolved in water or molten, salts conduct electricity due to the presence of free ions.
Melting and Boiling Points: Salts typically have high melting and boiling points due to the strong ionic bonds between their constituent ions.
Color: While many salts are colorless (e.g., sodium chloride), some can have distinct colors. For example, copper sulfate (CuSO4) is blue, and potassium dichromate (K2Cr2O7) is orange.
Taste: Many salts have a characteristic salty taste, although tasting chemicals is not recommended in a laboratory setting.
Hygroscopicity: Some salts, like calcium chloride (CaCl2), are hygroscopic, meaning they absorb moisture from the air.
Common Salts and Their Uses
Salts have numerous applications in our daily lives and various industries. Here’s a table showcasing some common salts and their uses:
Salt | Chemical Formula | Common Uses |
---|---|---|
Sodium Chloride (Table Salt) | NaCl | Food seasoning, de-icing roads, water softening |
Sodium Bicarbonate (Baking Soda) | NaHCO3 | Baking, cleaning, antacid |
Calcium Carbonate | CaCO3 | Antacid, dietary supplement, construction material |
Potassium Nitrate | KNO3 | Fertilizer, fireworks, food preservative |
Copper Sulfate | CuSO4 | Fungicide, algaecide, electrical plating |
Ammonium Chloride | NH4Cl | Batteries, soldering flux, cough medicine |
Magnesium Sulfate (Epsom Salt) | MgSO4 | Bath salt, soil amendment, medical treatments |
Silver Nitrate | AgNO3 | Photography, mirror production, medical uses |
pH of Salt Solutions
The pH of a salt solution can vary depending on the nature of the salt. This property is closely related to the concept of hydrolysis, which is the reaction of a salt with water. The pH of a salt solution can be:
Neutral (pH = 7): Salts formed from strong acids and strong bases, like sodium chloride (NaCl), produce neutral solutions.
Acidic (pH < 7): Salts formed from strong acids and weak bases, like ammonium chloride (NH4Cl), produce acidic solutions.
Basic (pH > 7): Salts formed from weak acids and strong bases, like sodium acetate (CH3COONa), produce basic solutions.
Understanding the pH of salt solutions is crucial in many applications, from agriculture to medicine.
Salt Hydrolysis
Salt hydrolysis is a process where a salt reacts with water, resulting in a solution that is not neutral. This process is particularly important for salts formed from weak acids or weak bases. Here’s a deeper look into the three types of salt hydrolysis:
Hydrolysis of Salts of Strong Acids and Weak Bases:
Example: NH4Cl (ammonium chloride)
NH4+ + H2O ⇌ NH3 + H3O+
This reaction produces excess H3O+ ions, resulting in an acidic solution.Hydrolysis of Salts of Weak Acids and Strong Bases:
Example: CH3COONa (sodium acetate)
CH3COO- + H2O ⇌ CH3COOH + OH-
This reaction produces excess OH- ions, resulting in a basic solution.Hydrolysis of Salts of Weak Acids and Weak Bases:
Example: NH4CH3COO (ammonium acetate)
Both the cation and anion undergo hydrolysis. The pH of the resulting solution depends on the relative strengths of the acid and base.
Buffer Solutions and Salts
Buffer solutions are an important application of salts in chemistry. A buffer solution resists changes in pH when small amounts of acid or base are added. Many buffer solutions consist of a weak acid and its conjugate base salt. For example:
- Acetic acid (CH3COOH) and sodium acetate (CH3COONa)
- Carbonic acid (H2CO3) and sodium bicarbonate (NaHCO3)
These buffer systems play crucial roles in maintaining pH balance in biological systems, such as blood in the human body.
Solubility Product Constant (Ksp)
The solubility product constant (Ksp) is an important concept related to the solubility of salts. It represents the equilibrium between a solid ionic compound and its ions in a saturated solution. For a salt AxBy that dissociates into x A^y+ cations and y B^x- anions, the Ksp is given by:
Ksp = [A^y+]^x [B^x-]^y
Where [A^y+] and [B^x-] are the molar concentrations of the ions at equilibrium.
Understanding Ksp is crucial for predicting precipitation reactions and calculating solubility of salts under various conditions.
Common Ion Effect
The common ion effect is a phenomenon where the solubility of a slightly soluble salt is decreased when a soluble compound with a common ion is added to the solution. This effect is based on Le Chatelier’s principle and has important applications in analytical chemistry and environmental science.
For example, the solubility of calcium carbonate (CaCO3) decreases in the presence of sodium carbonate (Na2CO3) due to the common carbonate ion.
Industrial Production of Salts
The industrial production of salts is a significant sector of the chemical industry. Here are some common methods used for large-scale salt production:
Solar Evaporation: Used for producing sea salt and other salts from brine.
Mining: Rock salt (halite) is often mined from underground deposits.
Solution Mining: Water is pumped into underground salt deposits, and the resulting brine is extracted and evaporated.
Neutralization Reactions: Many salts are produced by reacting acids with bases on an industrial scale.
Ion Exchange: Used for producing high-purity salts and in water treatment processes.
Environmental Impact of Salts
While salts are essential for life and have numerous industrial applications, their environmental impact must be considered:
Soil Salinity: Excessive salt accumulation in soil can lead to reduced crop yields and soil degradation.
Water Quality: High salt concentrations in freshwater bodies can harm aquatic ecosystems.
Road Salt: While effective for de-icing, road salt can contaminate groundwater and damage vegetation.
Desalination: The process of removing salt from seawater produces highly concentrated salt brine as a by-product, which can harm marine ecosystems if not properly managed.
Salts in Biochemistry
Salts play crucial roles in biochemical processes:
Electrolyte Balance: Salts like sodium chloride and potassium chloride are essential for maintaining proper electrolyte balance in living organisms.
Enzyme Activity: Many enzymes require specific salt concentrations for optimal activity.
Osmotic Pressure: Salts contribute to the osmotic pressure of biological fluids, crucial for cell function.
Signal Transduction: Some salts, particularly those containing calcium ions, play key roles in cellular signaling processes.
Future Perspectives in Salt Research
As our understanding of salts continues to evolve, several areas of research show promise:
Novel Materials: Researchers are exploring new salt-based materials for applications in energy storage, catalysis, and advanced materials.
Sustainable Agriculture: Developing salt-tolerant crops and improved soil management techniques to address soil salinity issues.
Green Chemistry: Finding more environmentally friendly alternatives to traditional salts in industrial processes.
Medical Applications: Exploring the potential of various salts in drug delivery systems and as therapeutic agents.
Desalination Technologies: Improving the efficiency and reducing the environmental impact of desalination processes.
Understanding salts is crucial not only for their direct applications but also for their role in broader chemical and biological processes. As we continue to explore the complexities of acid-base chemistry, the study of salts remains a fundamental and ever-evolving field, bridging the gap between theoretical chemistry and real-world applications.
Acids, bases, and salts form the cornerstone of chemistry, playing crucial roles in countless chemical reactions and everyday applications. From the tangy taste of citrus fruits to the cleaning power of household products, these substances are integral to our daily lives. Understanding their properties and interactions is essential for anyone interested in chemistry or seeking to grasp the fundamental principles that govern the world around us.
As we’ve explored in this post, each of these chemical categories has unique characteristics and uses. By recognizing the differences between acids, bases, and salts, we can better appreciate their importance in various fields, from industry to medicine. Whether you’re a student, a professional, or simply curious about chemistry, continue to explore these fascinating compounds and their impact on our world. The more we understand about acids, bases, and salts, the better equipped we are to harness their potential and address challenges in science and technology.
Enhance your understanding of acid-base chemistry with our 200-question worksheet. Covers pH, neutralization, indicators, and more. Perfect for students and chemistry enthusiasts.